We see far greater risk of massive irreversible sea level rise (SLR) at 2°C, on a scale of 12–20 meters or more in the long term. The climate record of the Earth over the pat few million years is quite clear:
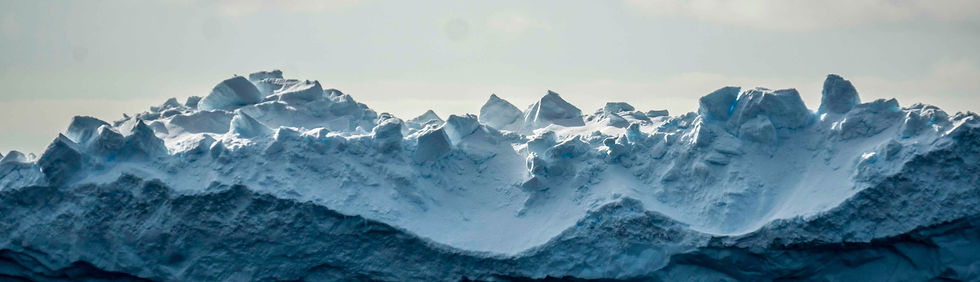
Sea Level Rise from Ice Sheets
We see far greater risk of massive irreversible sea level rise (SLR) at 2°C, on a scale of 12–20 meters or more in the long term. The climate record of the Earth over the pat few million years is quite clear:
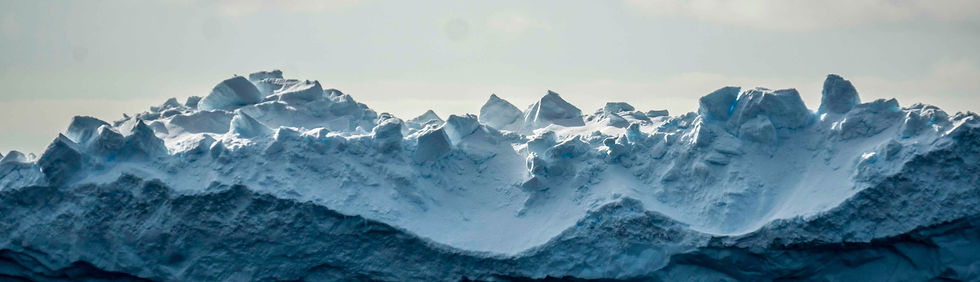
Sea Level Rise from Ice Sheets

Polar Ocean Acidification
Fish stocks such as cod are much more negatively affected by changes in the polar oceans at 2°C global warming than at 1.5°C global warming. These changes include ocean acidification, warmer and less salty sea water from increased river runoff, glacier melt and ice sheet melt; as well as greater competition from mid-latitude species moving polewards. In contrast, polar species and ecosystems have nowhere further to migrate.
​
Today’s rates of ocean acidification are greater than at any time in 3 million years, and pose an immediate and serious threat in cold polar waters, which absorb CO2 more quickly. The oceans will need 50–70,000 years to return to normal pH levels, a key argument for keeping CO2 levels as low as possible and against schemes aiming to decrease solar radiation rather than CO2.
Scientific Editors
Nina Bednaršek, Southern California Coastal Water Research Project
Helen Findlay, Plymouth Marine Laboratory
​
Scientific Reviewers
Richard Bellerby, East China Normal University/Norwegian Institute for Water Research
Sam Dupont, University of Gothenburg
Humberto E. González, University Austral of Chile/Fondap IDEAL
Peter Thor, Swedish Meteorological and Hydrological Institute (SMHI)
Paul Wassmann, UiT – The Arctic University of Norway
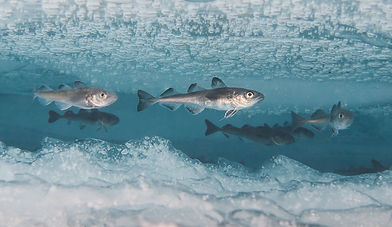
Arctic Cod. Image: Peter Leopod
Rapid Acidification
Increasing CO2 concentrations lead not only to climate change, but also to increasing rates of acidification of the world’s oceans. In addition to providing valuable food and other resources, the ocean provides a vital but less visible service to the global climate system by absorbing CO2 and limiting global warming, despite sharp increases in human carbon emissions. However, this carbon absorption comes with a price: when dissolved into seawater, CO2 forms carbonic acid. This phenomenon is known as ocean acidification; and its levels today are higher than at any point in the past three million years.
​
Ocean acidification is more severe in cold-water ocean environments, which absorb CO2 more quickly. The Southern Ocean (around Antarctica), the Arctic Ocean, and associated high-latitude seas are home to important fisheries; and are acidifying far more quickly than any other oceans on the planet.
Present and Projections
Since pre-industrial times, as human emissions of CO2 have grown, global average acidification levels have increased by 30%; with atmospheric CO2 now above 400 ppm and temperatures about 1°C above pre-industrial. In some parts of the polar oceans however, acidification has increased by 40% or more, fundamentally changing polar ocean chemistry.
Global temperatures peaking at 1.5°C will occur at atmospheric CO2 levels of around 450 ppm, which scientists of the Inter-academy Panel (a consortium of national Academies of Sciences) identified in 2009 as an upper boundary for global ocean acidification. This represents an additional 30% increase in acidification globally, with higher levels again projected in polar waters. However, by 2°C CO2 levels will have reached around 550 ppm; acidity will have increased by nearly 100% globally from pre-industrial times, and more than doubled in polar oceans.

Melt pools atop sea ice in the Arctic Ocean in August 2009.
Image: Pablo Clemente-Colon / National Ice Center
Corrosive Shell-Building Conditions
at Higher Emissions

Difference between acidification conditions in a 1.5° world (RCP2.6) (lower map), and a 4° world (RCP8.5) (upper map) by 2100. Red shows “undersaturated aragonite conditions,” a measure of ocean acidification meaning that shelled organisms will have difficulty building or maintaining their shells, leading to potential decline of populations and dietary sources for fish, with loss of biodiversity towards simplified food webs.
IPCC SROCC (2019)
Corrosive Waters
​
Ocean acidification dissolves the minerals that marine animals need to make their shells and structures, such as a coral’s skeleton. As the seawater becomes “corrosive” due to CO2, these shell-building minerals break down. In this way, ocean acidification harms the ability of organisms such as snails, urchins, clams, and crabs to build and maintain their shells. Acidification also challenges nonshell-building organisms, preventing them from growing and reproducing normally.
​
Critical Threshold
Atmospheric CO2 levels above 510 ppm, which will be passed sometime between 1.5°C and 2°C, are projected to cause widespread areas of corrosive waters in the Southern Ocean for these shell-building species. The Arctic Ocean appears to be even more sensitive: it likely has large regions of corrosive waters already seasonally at atmospheric CO2 levels between 400 and 450 ppm, well within even the 1.5°C boundary. Indeed, the world is at 410 ppm today, and shell damage has been observed for several years now in some regions of the polar oceans where acidification thresholds have been exceeded already due to local conditions. These corrosive areas began expanding in the Arctic Ocean in the 1990s.
In other words, because of their greater sensitivity, polar oceans already appear to be nearing a critical ocean acidification chemical threshold. There is high likelihood that these changes are a harbinger of much worse to come, due to CO2 already in the atmosphere continuing to be absorbed in coming years and decades, until, and unless, CO2 levels begin to fall sharply. In the Southern Ocean, the ability of some vulnerable organisms to build shells declined by around 4% between 1998 and 2014.
Pteropods – tiny marine snails known as “sea butterflies” – are particularly susceptible to ocean acidification and these expanding corrosive waters, with some shell damage observed today in portions of the Gulf of Alaska, Bering and Beaufort seas; as well as in portions of the Southern Ocean. Pteropods are hugely important in the polar food web, serving as an important source of food for young salmon, cod and other species.
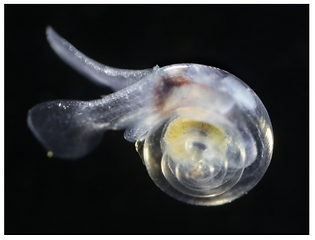
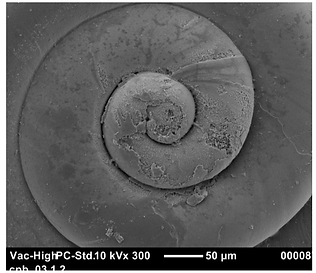
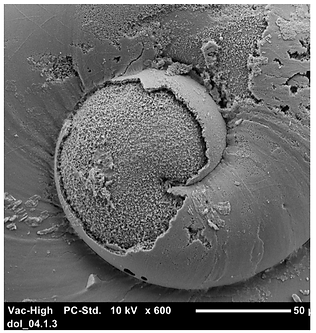
Top: Healthy living pteropod
Middle: Observed moderate shell damage (Arctic)
Bottom: Observed severe shell damage (Arctic)
Images: Dr. Nina Bednaršek.

Unprecedented Rates of Change
Global ocean acidity has been relatively stable over the past several million years. Almost all marine life today, including that of the polar oceans evolved to live in this relatively narrow band of ocean chemistry. Although some older marine organisms, such as horseshoe crabs managed to survive changes equivalent to those occurring today in the geologic past, those changes always took place over many thousands of years.
Today’s rate of change is unprecedented however in at least the past 65 million years, when severe oceans changes, including acidification occurred, resulting in the mass extinction of many shelled organisms. The speed of today’s acidification is therefore a key part of its threat: it is simply occurring far too quickly to allow many species to evolve and survive.
Irreversible Change
The chemical process of ocean acidification is well understood. The difficult reality for polar ecosystems is that the exact threshold for serious impacts from higher CO2 concentrations on marine organisms and ecosystems, as compared to these laboratory studies, will not really be known until they actually occur: first in isolated regions and with early-warning vulnerable species, such as the sea butterflies of some Arctic and Antarctic waters noted above; but then spreading to more regions and species.
At that point however, there is no known way for humans to reverse this damage, because these more acidic conditions will then persist for many thousands of years. This is because processes that buffer (remove) the acidity from the ocean occur very slowly, over nearly geologic time scales. Although CO2 “only” lasts for 800–1000 years in the atmosphere, it will take several thousands of years before ocean acidification levels begin to decline. Indeed, tens to hundreds of thousands of years will be necessary to bring acidification and its impacts back to pre-industrial levels, following the weathering of rocks on land into the ocean.
This very long lifetime of acidification in the oceans is one reason why mitigation efforts focused on “solar-radiation management,” as opposed to decreasing atmospheric CO2 represent a special threat to the health of the world’s oceans, especially those at the poles.
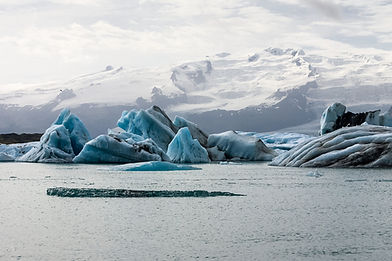
Warming Waters
The impacts of acidification come as both the polar oceans are warming at increasing rates, with marine “heat waves” occurring further polewards, with greater intensity and more frequently. The Southern Ocean has warmed more than many other ocean regions, and seems increasingly important in overall global ocean heat increase.
Over large areas of the seasonally ice-free Arctic, summer surface water temperatures have increased by around 0.5°C per decade since 1982, primarily due to sea ice loss and an inflow of ocean heat from lower latitudes beginning in the 2000s. Future projected Arctic warming will result in continued loss of sea ice, increased river run-off into the oceans and freshening of seawater.
Warming waters result in a poleward movement of other species, decreasing the ranges of polar species as they face increased competition for food resources. In some instances, especially where extreme heatwaves occur in the ocean, polar species have apparently even experienced lethal temperatures. Large die-offs of seabirds and gray whales in regions of the Bering Sea in summer 2019 seem to be associated with these marine heatwaves.
Freshening Waters
The freshening of polar oceans represents an additional stress for polar species. Polar oceans already have a lower salinityfrom freshwater coming off melting sea and land ice, but this incursion of freshwater will increase as the ice sheets, local glaciers and permafrost melt as temperature rises. Ice-associated algae (plants) and animals also are being lost as sea ice declines due to warming.
Therefore the loss of Arctic sea ice, especially thicker multi-year ice, which served as a kind of “polar ice reef” on which many species built their existence, further stresses the polar food chain.
​
The projected effects of climate-induced stressors on polar marine ecosystems present risks for commercial and subsistence fisheries, with implications for regional economies, cultures and the global supply of fish and shellfish.
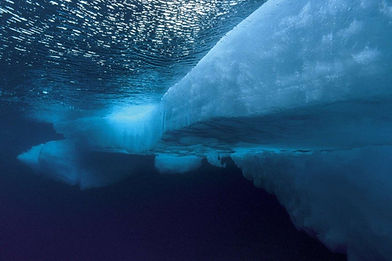
Under the Arctic sea ice. Image: Paul Nicklen
Threat of Extinction
The bottom line is that ocean acidification will have earlier and greater impacts on polar ecosystems and organisms; with even more, some potentially irreversible, occurring at higher temperatures and atmospheric CO2 concentrations.
This increased acidification also comes at the same time that polar species face other threats from climate change such as warming waters, ice-loss and freshening due to increased river run-off and ice melt.
There is no doubt that intense acidification in the gradient between CO2 levels today, through 1.5°C and beyond 2°C risks severe negative impacts for a range of polar marine organisms, from the smallest plankton to the largest fish, with severe consequences for polar ecosystems, fisheries and aquaculture.
The only known way to slow the process of polar ocean acidification is through cutting the amount of CO2 entering the atmosphere, aiming to hold levels within the 450 ppm range associated with warming of 1.5°C.
Learn more:
IPCC Special Report: Global Warming of 1.5°C
​
IPCC Special Report on the Ocean and Cryosphere in a Changing Climate
​
ICCI The Cryosphere1.5° Report
References
AMAP (2013) AMAP Assessment 2013: Arctic Ocean Acidification. Arctic Monitoring and Assessment Programme (AMAP), Oslo, Norway. viii + 99 pp.
Baumann H, Talmage SC, Gobler CJ (2012) Reduced early life growth and survival in a fish in direct response to increased carbon dioxide. Nature Climate Change, 2: 38–41, doi:10.1038/nclimate1291
Bednaršek N, Tarling GA, Bakker DCE, Fielding S, Feely RA (2014) Dissolution dominating calcification process in polar pteropods close to the point of aragonite undersaturation.
PLoS ONE, 9: e109183, doi:10.1371/journal.pone.0109183
Bednaršek N, Feely RA, Reum JCP, Peterson B, Menkel J, Alin SR, Hales B (2014) Limacina helicina shell dissolution as an indicator of declining habitat suitability owing to ocean
acidification in the California Current Ecosystem. Proceedints of the Royal Society B: Biological Sciences, 281: 20140123,doi:10.1098/rspb.2014.0123
Cummings V et al. (2011) Ocean acidification at high latitudes: potential effects on functioning of the Antarctic bivalve Laternula elliptica. PLoS ONE, 6.1: e16069, doi:10.1371/journal.pone.0016069
Dupont S & Pörtner H (2013) Get ready for ocean acidification. Nature, 498: 429, doi:10.1038/498429a
Dupont S, Havenhand J, Thorndyke W, Peck L & Thorndyke M (2008) Near-future level of CO2-driven acidification radically affects larval survival and development on the brittle star
Ophiothrix fragilis. Marine Ecology Progress Series, 373: 285-294, doi: 10.3354/meps07800
Gattuso JP, Magnan A, Billé R, Cheung WWL, Howes EL, Joos F, Allemand D, Bopp L, Cooley SR, Eakin CM, Hoegh-Guldberg O, Kelly RP, Pörtner HO, Rogers AD, Baxter JM, Laffoley D, Osborn D, Rankovic A, Rochette J, Sumaila UR, Treyer S & Turley C (2015) Contrasting futures for ocean and society from different anthropogenic CO2 emissions scenarios. Science, 349: aac4722, doi:10.1126/science.aac4722.
IAP (2009) IAP Statement on Ocean Acidification. Retrieved
from: http://www.interacademies.net/File.aspx?id=9075
IPCC (2014) Climate Change 2014: Impacts, Adaptation, and Vulnerability. Part A: Global and Sectoral Aspects. Contribution of Working Group II to the Fifth Assessment Report of the Intergovernmental Panel on Climate Change [Field, C.B., V.R. Barros, D.J. Dokken, K.J. Mach, M.D. Mastrandrea, T.E. Bilir, M. Chatterjee, K.L. Ebi, Y.O. Estrada, R.C. Genova, B. Girma, E.S. Kissel, A.N. Levy, S. MacCracken, P.R. Mastrandrea, and L.L. White (eds.)]. Cambridge University Press, Cambridge, United Kingdom and New York, NY, USA, 1132 pp.
IPCC (2014) Climate Change 2014: Impacts, Adaptation, and Vulnerability. Part B: Regional Aspects. Contribution of Working Group II to the Fifth Assessment Report of the Intergovernmental Panel on Climate Change [Barros, V.R., C.B. Field, D.J. Dokken, M.D. Mastrandrea, K.J. Mach, T.E. Bilir, M. Chatterjee, K.L. Ebi, Y.O. Estrada, R.C. Genova, B. Girma, E.S. Kissel, A.N. Levy, S. MacCracken, P.R. Mastrandrea, and L.L. White (eds.)].
Cambridge University Press, Cambridge, United Kingdom and New York, NY, USA, 688 pp.
IPCC (2018) Global warming of 1.5°C. An IPCC Special Report on the impacts of global warming of 1.5°C above pre-industrial levels and related global greenhouse gas emission pathways, in the context of strengthening the global response to the threat of climate change, sustainable development, and efforts to eradicate poverty [V. Masson-Delmotte, P. Zhai, H. O. Pörtner, D. Roberts, J. Skea, P.R.Shukla,A. Pirani, W. Moufouma-Okia, C.Péan, R. Pidcock, S. Connors, J. B. R. Matthews, Y. Chen, X. Zhou, M. I. Gomis, E. Lonnoy, T. Maycock, M. Tignor, T. Waterfield(eds.)].
IPCC (2019) Special Report on the Oceans and Cryosphere in a Changing Climate [H.-O. Pörtner, D.C. Roberts, V. Masson-Delmotte, P. Zhai, M. Tignor, E. Poloczanska, K. Mintenbeck, M. Nicolai, A. Okem, J. Petzold, B. Rama, N. Weyer (eds.)]. In press.
Mathis JT, Cross JN, Evans W & Doney SC (2015) Ocean acidification in the surface waters of the Pacific-Arctic boundary regions. Oceanography, 28(2):122–135, doi:10.5670/oceanog.2015.36
McNeil BI, Matear RJ (2008) Southern Ocean acidification: A tipping point at 450-ppm atmospheric CO2. Proceedings of the National Academy of Sciences, 105(48): 18860-18864, doi:10.1073/pnas.0806318105
Riebesell,U. & Gattuso JP (2015) Lessons learned from ocean acidification research. Nature Climate Change, 5: 12–14, doi:10.1038/nclimate2456
Sasse TP, McNeil BI, Matear RJ, Lenton A (2015) Quantifying the influence of CO2 seasonality on future aragonite undersaturation onset. Biogeosciences, 12: 6017-6031, doi:10.5194/bg-12-6017-2015
Wittmann AC & Pörtner HO (2013) Sensitivities of extant animal taxa to ocean acidification. Nature Climate Change, 3: 995-1001, doi:10.1038/nclimate1982